Last updated: Wednesday, January 26, 2005
The skin
Skin incisions
Rotator cuff surgery can usually be accomplished through cosmetically acceptable incisions in the lines of the skin (see figure 1). These skin lines run obliquely from superior lateral to infero medial. The usual "superior" approach to the cuff is made through such an oblique incision which runs over the anterior corner of the acromion.

Figure 1
The deltoid muscle
The deltoid arises from the lateral half of the clavicle, the acromion and posteriorly from the scapular spine.
About the deltoid
The origin of the deltoid is over the entire anterior height of the acromion, thus in the performance of an acromioplasty a substantial amount of the deltoid origin must be detached, whether the procedure is performed open or arthroscopically. (Torpey, McFarland, 1996) The deltoid has an important and constant tendon of origin separating its anterior and lateral thirds. This tendon attaches to the anterior lateral corner of the acromion in which location it provides the key to the anterior deltoid-splitting approach to the cuff. By making the deltoid split down the center of the tendon, the surgeon can be assured of having strong tendinous "handles" on the muscle for use in deltoid closure (see figure 1). While it is often said that the location of the axillary nerve is on the average five centimeters distal to the acromion, its anterior branches swoop upwards so that it is desirable to limit the inferior extent of the deltoid split to minimize the risk of injury to the axillary nerve branches (see figure 1).
The acromion, the coracoid and the coracoacromial
The acromion is a scapular process arising from three separate centers of ossification--a preacromion, a mesoacromion, and a meta-acromion. (Chung and Nissenbaum, 1975, Mudge, Wood, 1984, Samilson, 1980)
Centers of ossification
These centers of ossification are usually united by age 22. When these centers fail to unite, the ununited portion is referred to as an os acromiale. This condition may have been first recognized by Schar and Zweifel (Schar and Zweifel, 1936) in 1936, as was mentioned by Pettersson. (Pettersson, 1942) Grant (Grant, 1972) found that 16 of 194 cadavers aged over 30 years demonstrated incomplete fusion of the acromion; the condition was bilateral in 5 subjects and unilateral in 11 subjects. In a large review of 1000 radiographs, Liberson (Liberson, 1937) found unfused acromia in 2.7 per cent; of these, 62 per cent were bilateral. Most commonly the lesion is a failure of fusion of the mesoacromion to the meta-acromion. He found the axillary view to be most helpful in revealing the condition. The size of the unfused fragment may be substantial, up to five by two centimeters. (Neer, 1972) Resection of a fragment this large creates a serious challenge for deltoid reattachment.
Norris and coworkers (Norris, Fischer, 1983) and Bigliani and associates (Bigliani, Norris, 1983) have pointed to an association of cuff degeneration and unfused acromial epiphysis. Mudge and coworkers (Mudge, Wood, 1984) found that 6 percent of 145 shoulders with cuff tears had an os acromiale; whereas Liberson found a 2.7 incidence of this finding in unselected scapulae. (Liberson, 1937) The statistical and clinical significance of this association remains unclear.
An additional anatomical feature of importance is the acromial branch of the thoracoacromial artery. This artery runs in close relation to the coracoacromial ligament and often is transected in the course of an acromioplasty and coracoacromial ligament section.
The coracoid arises from two or three ossification centers. (Samilson, 1980). It provides the medial attachment site for both the coracohumeral and coracoacromial ligament. In that their muscle bellies lie medial to it, the neighboring supraspinatus and subscapularis tendons must be able to glide by the coracoid with their full excursion during shoulder movement. Scarring of one or both these tendons to the coracoid can inhibit passive and active shoulder motion. While the coracoid does not normally contact the anterior subscapularis tendon, forced internal rotation, particularly in the presence of a tight posterior capsule, can produce such contact due to obligate translation. (Gerber, Terrier, 1985, Harryman, Sidles, 1990)
The coracoacromial ligament spans from the undersurface of the acromion to the lateral aspect of the coracoid and is continuous with the less dense clavipectoral fascia. It forms a substantial part of the superficial aspect of the humeroscapular motion interface (see figures 2 and 3). This ligament may be thought of as the spring ligament of the shoulder, maintaining the normal relationships between the coracoid and the acromion. Separation of these two scapular processes has been observed on sectioning this ligament in cadavers. (Flatow, Raimondo, 1996)
The coracoacromial arch is the inferiorly concave smooth surface consisting of the anterior undersurface of the acromion and coracoacromial ligament. It provides a strong ceiling for the shoulder joint along which the cuff tendons must glide during all shoulder movements (see figures 2 and 3). Passage of the cuff tendons and proximal humerus under this arch is facilitated by the subacromial-subdeltoid bursa, which normally is not a space, as is often shown on diagrams, but rather two serosal surfaces in contact with each other, one on the undersurface of the coracoacromial arch and deltoid and the other on the cuff. These sliding surfaces are lubricated by bursal surfaces and synovial fluid.
The recognition of the gliding articulation between the arch and the cuff is not new. Renoux et al credited Ludkewitch, who in 1900 recognized that the proper functioning of the "scapulohumeral articulation" requires the presence of a "secondary socket" which extends the glenoid fossa of the scapula above, in front and behind, and of which the coracoacromial arch forms the ceiling. (Renoux, Monet, 1986) In 1934 Codman stated that the coracoacromial arch was an auxiliary joint of the shoulder and that its roughly hemispheric shape was "almost a counterpart in the size and curvature of the articular surface of the true joint." He referred to the "gleno-coraco-acromial socket". (Codman, 1934b) His belief in the importance of the coracoacromial arch was great enough to state that "the coracoacromial ligament has an important duty and should not be thoughtlessly divided at any operation." Wiley has pointed to the severe superior instability when the ceiling of the shoulder is lost in association with cuff deficiency. (Wiley, 1991) Kernwein et al in 1961 stressed the importance of the "suprahumeral gliding mechanism" consisting of the coraco-acromial arch on one side and the rotator cuff and biceps tendon on the other separated by the subacromial bursa. ( Kernwein, Roseberg, 1961) They believed that these two opposing, gliding surfaces and interposed bursa constituted a fifth joint which contributed to shoulder motion. DePalma, in 1967, also recognized the intimate relationship between the arch and the structures below it. (DePalma, 1967) He referred to the arch, together with the head of the humerus, the rotator cuff and subacromial bursa, as the "superior humeral articulation."
Matsen and Romeo described the humeroscapular motion interface as an articulation (see figures 2 and 3) between the cuff, humeral head and biceps on the inside and the coracoacromial arch, deltoid and coracoid muscles on the outside (Matsen, Lippitt, 1994) and measured up to 4 cm of gliding at this articulation in normal shoulders in vivo.
Recent investigations (Burns and Whipple, 1993, Flatow, Raimondo, 1996, Flatow, Soslowsky, 1994, Regan and Richards, 1989, Wuelker, Plitz, 1994, Ziegler, Matsen III, 1996) have pointed to the importance of contact and load transfer between the rotator cuff and the coracoacromial arch in the function of normal shoulders, including the provision of superior stability. Because there is normally no gap between the superior cuff and the coracoacromial arch, the slightest amount of superior translation compresses the cuff tendon between the humeral head and the arch. Superior displacement is opposed by a downward force exerted by the coracoacromial arch through the cuff tendon to the humeral head. Ziegler et al (Ziegler, Matsen III, 1996) demonstrated this "passive resistance" effect in cadavers by showing that the acromion bent upwards when a superiorly directed force was applied to the humerus in the neutral position. The amount of acromial deformation was directly related to the amount of superior force applied to the humerus; the load being transmitted through the intact superior cuff tendon. Furthermore these authors found that the amount of superior humeral displacement resulting from a superiorly directed humeral load of 80 N was increased from 1.7 mm to 5.4 mm when the cuff tendon was excised (p < .0001) (see figures 4 and 5). These results indicate that
- the intact superior cuff tendon is subject to compressive loading between the humeral head and the coracoacromial arch and
- that the presence of this tendon provides passive resistance against superior displacement of the humeral head when superiorly directed loads are applied.
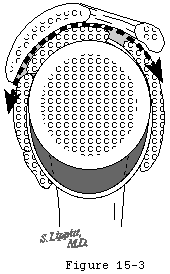
Figure 2

Figure 3

Figure 4

Figure 5
Flatow et al (AAOS 1996) also noted that, in a dynamic cadaver model, the presence of the supraspinatus tendon limited superior translation of the humeral head, even when there was no tension in the tendon from simulated muscle action.
The spacer effect of the superior cuff tendon is evident in comparing shoulders with intact cuffs (see figure 6) with those in which the superior tendon is deficient (see figure 7).
Both Ziegler et al and Flatow et al cautioned that the superior stability of the shoulder is dependent on an intact coracoacromial arch. Surgical sacrifice of the arch can lead to severe superior stability.
Changes in the coracoacromial arch have been described in association with cuff disease (Soslowsky, An, 1994 July) along with variations of acromial shape.(Bigliani, Norris, 1983, Neer, 1972) Bigliani and colleagues (Bigliani, Morrison, 1986) studied 140 shoulders in 71 cadavers. The average age was 74.4 years. They identified three acromial shapes: Type I (flat) in 17 per cent, Type II (curved) in 43 per cent, and Type III (hooked) in 40 per cent. Fifty-eight per cent of the cadavers had the same type of acromion on each side. Thirty-three per cent of the shoulders had full-thickness tears, of which 73 per cent were seen in the presence of Type III acromia, 24 per cent in Type II, and 3 per cent in Type I. The anterior slope of the acromion in shoulders with cuff tears averaged 29 degrees, slightly more than the slope of those without cuff tears which averaged 23 degrees. The clinical significance of this relatively small difference is not known. A number of other authors have reported that patients with cuff defects are more likely to have hooked or angled acromia. (Morrison and Bigliani, 1987, Toivonen, Tuite, 1995, Tuite, Toivonen, 1995) Nicholson et al (Nicholson, Goodman, 1996) demonstrated on a review of 420 scapulas that spur formation of the anterior acromion was an age-related process such that individuals under age of 50 had less 1/4 the prevalence of those over 50 years of age. The status of the cuffs of these shoulders is unknown.
Although these data indicate a strong association between aging, the presence of cuff tears, and alterations of acromial contour, it has been unclear whether the change in acromial shape was caused by or resulted from the cuff defect, or whether both were consequences of aging. As pointed out by Neer, (Neer, 1972) disease of the rotator cuff causes characteristic changes on the undersurface of the coracoacromial arch. In a remarkable study, Ozaki et al (Ozaki, Fujimoto, 1988) correlated the histology of the acromial undersurface with the status of the rotator cuff in 200 cadaver shoulders. Cuff tears which did not extend to the bursal surface were associated with normal acromial histology, whereas those which extended to the bursal surface were associated with changes in pathological changes in the acromial undersurface. They concluded that most cuff tears are related to tendon degeneration and that acromial changes are secondary to pathology of the bursal side of the cuff. These results are similar to those reported Fukuda et al. (Fukuda, Hamada, 1990)
Recent studies suggest that type II and III acromia are acquired, rather than being developmental. (Yazici, Kapuz, 1995) In that most acromial "hooks" lie within the coracoacromial ligament (see figure 8), it seems likely that they are actually traction spurs in this ligament (analogous to the traction spur seen in the plantar ligament at its attachment to the calcaneus) (see figure 9). The traction loads producing this hook may result from loading of the arch by the cuff and may be increased with increasing dependency on the coracoacromial arch for superior stability in the presence of cuff degeneration. (Flatow, Raimondo, 1996, Flatow, Soslowsky, 1994, Ziegler, Matsen III, 1996) The concept of the "hook" as a traction phenomenon was first forwarded by Neer over 25 years ago. (Neer, 1972) More recently, Putz and Reichelt (Putz and Reichelt, 1990) reported that three quarters of 133 operative specimens of the coracoacromial ligament showed chondroid metaplasia near the acromial insertion, suggesting that this metaplastic area becomes the acromial "hook" by enchondral bone formation. (Ogata and Uhthoff, 1990) Because this "hook" lies within the ligament and points toward the coracoid (see figure 8), it seems unlikely that it would jeopardize the passage of the cuff beneath the coracoacromial arch (see figure 9). Even in the severest cases of cuff tear arthropathy, the undersurface of the coracoacromial arch commonly presents a smooth articulating concavity (see figures 9 and 10).
In view of the forgoing, it is instructive to consider the humeroscapular articulation as consisting of two concentric spheres, the humeral head sphere and the sphere represented by the inferior surface of the coracoacromial arch. Together these two spheres enhance both shoulder stability and the surface available for scapulohumeral load transfer (see figure 11). (Codman, 1934b) Normally, the spheres of the humeral head and coracoacromial arch share the same center. The difference in radius of the two spheres (R and r in figure 11) is provided by the thickness of the rotator cuff which serves as a spacer. In the presence of posterior capsular tightness, shoulder flexion and/or internal rotation cause obligate anterosuperior translation of the humeral head and loss of the concentricity of the two spheres (see figure 12). (Cofield and Simonet, 1984, Harryman, Sidles, 1990) As a result, the convex cuff-covered head is forced against the anterior undersurface of the concave coracoacromial arch, rather than rotating concentrically beneath it (see figure 11). In the presence of degeneration of the cuff tendon, the shoulder may lose the concentricity of the humeral head and coracoacromial arch spheres (see figure 13). Taken together, these observations reinforce the shoulder's need for
- normal posterior capsular laxity,
- a smooth, concentric and congruent coracoacromial undersurface, and
- a normally thick and uniform cuff interposed between the humeral head and coracoacromial arch.
The acromioclavicular joint. Osteophytes from the acromioclavicular joint may encroach on the space normally occupied by the cuff tendons (see figure 14). In a series of 47 patients with arthrographically confirmed supraspinatus tendon ruptures, Peterson and Gentz (Peterson and Gentz, 1983) found that 51 per cent had distally pointing acromioclavicular joint osteophytes. A similar incidence was found in their series of 170 autopsy specimens with cuff defects. The incidence of distally pointing acromioclavicular osteophytes in normal shoulders was 14 per cent and 10 per cent in the clinical and cadaver studies, respectively. It is recognized, however, that degenerative changes in the cuff and degenerative changes in the acromioclavicular joint may coexist in the aging population without the former being causally related to the latter. It is furthermore recognized that acromioclavicular osteophytes may be sufficiently medial that they not jeopardize the cuff.
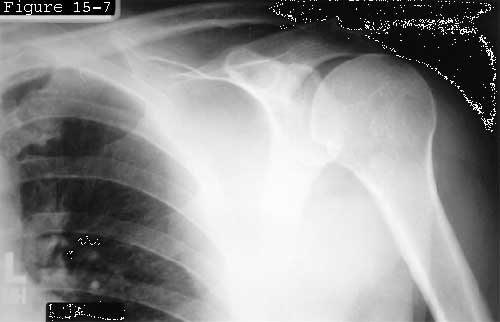
Figure 6

Figure 7

Figure 8

Figure 9

Figure 10

Figure 11

Figure 12

Figure 13

Figure 14
The rotator cuff
The rotator cuff is the complex of four muscles that arise from the scapula and whose tendons blend in with the subjacent capsule as they attach to the tuberosities of the humerus.
Anatomy of rotator cuff
The subscapularis arises from the anterior aspect of the scapula and attaches over much of the lesser tuberosity. It is innervated by the upper and lower subscapular nerves. (Yung and Harryman, 1995) The supraspinatus muscle arises from the supraspinatus fossa of the posterior scapula, passes beneath the acromion and the acromioclavicular joint, and attaches to the superior aspect of the greater tuberosity. It is innervated by the suprascapular nerve after it passes through the suprascapular notch. The infraspinatus muscle arises from the infraspinous fossa of the posterior scapula and attaches to the posterolateral aspect of the greater tuberosity. It is innervated by the suprascapular nerve after it passes through the spinoglenoid notch. The teres minor arises from the lower lateral aspect of the scapula and attaches to the lower aspect of the greater tuberosity. It is innervated by a branch of the axillary nerve.
Tendons
The insertion of these tendons as a continuous cuff around the humeral head (see figure 15) permits the cuff muscles to provide an infinite variety of moments to rotate the humerus and to oppose unwanted components of the deltoid and pectoralis muscle forces. For an excellent review of the anatomy and histology of the rotator cuff, the reader is referred to the works of Clark and Harryman (Clark, 1988, Clark and Harryman II, 1992, Clark, Sidles, 1990) and Warner's chapter on shoulder anatomy in The Shoulder: A Balance of Mobility and Stability. (Warner, 1993)
The long head of the biceps tendon may be considered a functional part of the rotator cuff. It attaches to the supraglenoid tubercle of the scapula, runs between the subscapularis and the supraspinatus, and exits the shoulder through the bicipital groove under the transverse humeral ligament, attaching to its muscle in the proximal arm. Slatis and Aalto (Slatis and Aalto, 1979) point out that the coracohumeral ligament and the transverse humeral ligament keep the biceps tendon aligned in the groove. Tension in the long head of the biceps can help compress the humeral head into the glenoid. Furthermore, this tendon has the potential for guiding the head of the humerus as it is elevated, the bicipital groove traveling on the biceps tendon like a monorail on its track. This mechanism helps to explain why the humerus is capable of substantial rotation when it is adducted and allows very little rotation when it is maximally abducted (in which position the tuberosities are constrained as they straddle the biceps tendon near its attachment to the supraglenoid tubercle).
Mechanics of cuff action
The mechanics of cuff action is complex. The humeral torque resulting from a cuff muscle's contraction is determined by the moment arm (the distance between the effective point of application of this force and the center of the humeral head) and the component of the muscle force which is perpendicular to it (see figure 16). (Wuelker, Wirth, 1995).
The magnitude of force deliverable by a cuff muscle is determined by its size, health, and condition as well as the position of the joint. The cuff muscles' contribution to shoulder strength has been evaluated by Colachis and associates, (Colachis and Strohm, 1971, Colachis, Strohm, 1969) who used selective nerve blocks and found that the supraspinatus and infraspinatus provide 45 per cent of abduction and 90 per cent of external rotation strength. Howell and coworkers (Howell, Imobersteg, 1986) measured the torque produced by the supraspinatus and deltoid muscles in forward flexion and elevation. They found that the supraspinatus and deltoid muscles are equally responsible for producing torque about the shoulder joint in the functional planes of motion. Other estimates of the relative contributions of the rotator cuff to shoulder strength have been published. (Bigliani, Morrison, 1986, Cofield, 1985, Van Linge and Mulder, 1963)
Factors of analysis
There are at least three factors which complicate the analysis of the contribution of a given muscle to shoulder strength:
- the force and torque that a muscle can generate varies with the position of the joint: muscles are usually stronger near the middle of their excursion and weaker at the extremes. (Lieber, 1992)
- the direction of a given muscle force is determined by the position of the joint. For example, the supraspinatus can contribute to abduction and/or external rotation, depending on the initial position of the arm. (Otis, Jiang, 1994)
- the effective humeral point of application for a cuff tendon wrapping around the humeral head is not its anatomic insertion, but rather is the point where the tendon first contacts the head, a point which usually lies on the articular surface (see figure 17).
Functions of cuff muscles
- They rotate the humerus with respect to the scapula.
- They compress the head into the glenoid fossa, providing a critical stabilizing mechanism to the shoulder, known as concavity compression. While in the past the cuff muscles were referred to as head depressors, it is evident that the inferiorly directed components of the cuff muscle force is small; instead the primary stabilizing function of the cuff muscles is through head compression into the glenoid (see figure 18). (Sharkey, Marder, 1994, Wuelker, Roetman, 1994)
- They provide muscular balance, a critical function which will be discussed in some detail here. In the knee, the muscles generate torques primarily about a single axis: that of flexion-extension. If the quadriceps pull is a bit off-center, the knee still extends. By contrast, in the shoulder, no fixed axis exists. In a specified position, activation of a muscle creates a unique set of rotational moments. For example, the anterior deltoid can exert moments in forward elevation, internal rotation, and cross-body movement (see figure 19). If forward elevation is to occur without rotation, the cross-body and internal rotation moments of this muscle must be neutralized by other muscles, such as the posterior deltoid and infraspinatus (see figure 20).(Sharkey, Marder, 1994) As another example, use of the latissimus dorsi in a movement of pure internal rotation requires that its adduction moment by neutralized by the superior cuff and deltoid. Conversely, use of the latissimus in a movement of pure adduction requires that its internal rotation moment be neutralized by the posterior cuff and posterior deltoid muscles.
The timing and magnitude of these balancing muscle effects must be precisely coordinated to avoid unwanted directions of humeral motion. For a gymnast to hold her arm motionless above her head, all the forces and torques exerted by each of her shoulder muscles must add up to zero. Thus the simplified view of muscles as isolated motors, or as members of force couples must give way to an understanding that all shoulder muscles function together in a precisely coordinated way: opposing muscles canceling out undesired elements leaving only the net torque necessary to produce the desired action. (Rowlands, Wertsch, 1995)
This degree of coordination requires a preprogrammed strategy of muscle activation or engram that must be established before the motion is carried out. The rotator cuff muscles are critical elements of this shoulder muscle balance equation. (Basmajian and Bazant, 1959, DePalma, 1967, Flanders, 1993, Grigg, 1993, Inman, Saunders, 1944, Jens, 1964, Joessel, 1880, Lieber and Friden, 1993, Saha, 1971, Speer and Garrett, 1993, Symeonides, 1972, Walker, Couch, 1987)

Figure 15
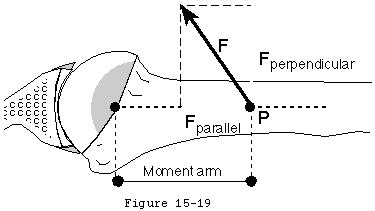
Figure 16
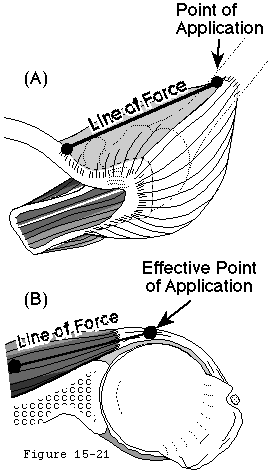
Figure 17

Figure 18

Figure 19

Figure 20
Vascular anatomy
The vascular anatomy of the cuff tendons has been described by a number of investigators (Brooks, Revell, 1992, Lohr and Uhthoff, 1990, Moseley and Goldie, 1963, Rathbun and Macnab, 1970, Rothman and Parke, 1965) Lindblom (Lindblom, 1939a, Lindblom, 1939b) described an area of relative avascularity in the supraspinatus tendon near its insertion. Rothman and Parke (Rothman and Parke, 1965) found contributions to the cuff vessels from the suprascapular, anterior circumflex, and posterior circumflex arteries in all cases. The thoracoacromial contributed in 76 per cent to the cuff's blood supply, the suprahumeral in 59 per cent, and the subscapular in 38 per cent. These authors found the area of the supraspinatus just proximal to its insertion to be markedly undervascularized in relation to the remainder of the cuff. Uhthoff and coworkers (Uhthoff, Loehr, 1986 Oct 27) observed relative hypovascularity of the deep surface of the supraspinatus insertion as compared with its superficial aspect.
By contrast, Moseley and Goldie studied the vascular pattern in the cuff tendons including the "critical zone" of the supraspinatus (i.e. the anterior corner of the tendon near its insertion which is prone to ruptures and calcium deposits). They found a vascular network which received contributions from the anterior humeral circumflex, the subscapular, and the suprascapular arteries. (Moseley and Goldie, 1963) They concluded that the critical zone was not much less vascularized than other parts of the cuff; rather, it was rich in anastomoses between the osseous and tendinous vessels. Rathbun and Macnab (Rathbun and Macnab, 1970) found that the filling of cadaveric cuff vessels was dependent on the position of the arm at the time of injection. They noted a consistent zone of poor filling near the tuberosity attachment of the supraspinatus when the arm was adducted; with the arm in abduction, however, there was almost full filling of vessels to the point of insertion. They suggested that some of the previous data suggesting hypovascularity was, in fact, due to this artifact of positioning. Nixon and DiStefano (Nixon and DiStefano, 1975) suggested that the "critical zone" of Codman corresponds to the area of anastomoses between the osseous vessels (the anterolateral branch of the anterior humeral circumflex and the posterior humeral circumflex) and the muscular vessels (the suprascapular and the subscapular vessels).
Recently, the vascularity of the supraspinatus tendon has been reconfirmed by the laser Doppler studies of Swiontkowski et al. (Swiontkowski, Iannotti, 1990) The laser Doppler assesses red cell motion at a depth of 1 to 2 mm. These investigators found substantial flow in the "critical zone" of normal tendon and increased flow at the margins of cuff tears. Furthermore, Clark (Clark and Harryman II, 1992) and Clark (Clark, 1988) and Clark et al (Clark, Sidles, 1990) found no avascular areas on his extensive histological studies of the supraspinatus tendon.
Uhthoff and Sarkar (Uhthoff and Sarkar, 1991b) examined biopsy specimens obtained during surgery on 115 patients with complete rotator cuff rupture. They found vascularized connective tissue covering the area of rupture and proliferating cells in the fragmented tendons. They concluded that the main source of fibrovascular tissue for tendon healing was the wall of the subacromial bursa.
Supraspinatus insertion
The histology of the supraspinatus insertion has been studied in some detail. Codman (Codman, 1934a) observed that there were "transverse fibers in the upper portion of the tendon." He stated that "the insertion of the infraspinatus overlaps that of the supraspinatus to some extent. Each of the other tendons also interlaces its fibers to some extent with its neighbor's tendons." In detailed anatomical studies, Clark and Harryman (Clark and Harryman II, 1992) and Clark (Clark, 1988) and Clark et al (Clark, Sidles, 1990) studied the tendons and capsule of the rotator cuffs from shoulders aged 17 to 72 years. They found that the tendons splayed out and interdigitated to form a common continuous insertion on the humerus. The biceps tendon was ensheathed by interwoven fibers derived from the subscapularis and supraspinatus. Blood vessels were noted throughout the tendons with no avascular zones. When dissecting what initially appeared to be an intact cuff, the authors frequently encountered a deep-substance tear in which fibers were avulsed from the humerus.
Benjamin and coworkers (Benjamin, Evans, 1986) have analyzed four zones of the supraspinatus attachment to the greater tuberosity:
- the tendon itself,
- uncalcified fibrocartilage,
- calcifiedfibrocartilage, and
- bone.
Whereas there were blood vessels in the other three zones, the zone of uncalcified fibrocartilage appeared avascular. A tidemark existed between the uncalcified and calcified fibrocartilage that was continuous with the tidemark between the uncalcified and calcified portions of articular cartilage. The collagen fibers often meet this tidemark approximately at right angles. In the tendon of the supraspinatus there was an abrupt change in fiber angle just before the tendon becomes fibrocartilaginous and only a slight change in angle within fibrocartilage. In interpreting the significance of these findings, these authors point out that the angle between the humerus and the tendon of the supraspinatus changes constantly in shoulder movement (see figure 35). While the belly of the muscle remains parallel to the spine of the scapula, the tendon must bend to reach its insertion. This bending appears to take place above the level of the fibrocartilage so that the collagen fibers meet the tidemark at right angles. The fibrocartilage provides a transitional zone between hard and soft tissues, protecting the fibers from sharp angulation at the interface between bone and tendon. The fibrocartilage pad keeps the tendon of the supraspinatus from rubbing on the head of the humerus during rotation, as well as keeping it from bending, splaying out, or becoming compressed at the interface with hard tissue.
Loading environment
The loading environment of the cuff tendon fibers is complex, even in the normal shoulder. These fibers sustain concentric tension loads when the humerus is moved actively in the direction of action of the cuff muscle (see figure 22). They sustain eccentric tension loads as they resist humeral motion or displacement in directions opposite the direction of action of the cuff muscles (see figure 23). The tendon fibers are subjected to bending loads when the humeral head rotates with respect to the scapula (see figure 21). As observed by Sidles (Matsen, Lippitt, 1994) in MRI scans positioned with the arm positioned at the limits of motion, the glenoid rim can apply a sheering load to the deep surface of the tendon insertion (see figure 24). This abutment of the labrum of the cuff against the cuff insertion may be a better explanation than acromial impingement for the deep surface cuff detects seen in throwers (see figures 25 and 26). (Ferrari, Ferrari, 1994, Jobe, 1995, Liu and Boynton, 1993, Rossi, Ternamian, 1994, Tirman, Bost, 1994, Walch, Liotard, 1991)

Figure 21

Figure 22

Figure 23

Figure 24

Figure 25

Figure 26
Recently Ziegler et al (Ziegler, Matsen III, 1996) suggested that the superior cuff tendon also experiences compressive loads as it is squeezed between the humeral head and the coracoacromial arch when superiorly directed loads are applied to the humerus. In a cadaver model, they found that the preponderance of an upward directed humeral load was transmitted through the cuff tendon to the overlying acromion. When the cuff tendon was excised the humeral head moved cephalad 6 millimeters until the superior humeral load was applied directly to the acromion (see figures 27 and 28). Using completely different methodologies Lazarus (Lazarus, Harryman II, 1995, February 16-21) and Poppen and Walker (Poppen and Walker, 1976) also found that the humeral head translated 6 millimeters superiorly when the cuff tendon was absent. Flatow et al (Flatow, Soslowsky, 1994) referred to this phenomenon as the spacer effect of the cuff tendon. Kaneko et al (Kaneko, DeMouy, 1995) found that superior displacement of the humeral head was one of the most significant plain radiographic signs of massive cuff deficiency (see figure 29). Sigholm and colleagues (Sigholm, Styf, 1988) found evidence of this normal tendon compression in vivo. Using a micropipette infusion technique, they found that the normal subacromial resting pressure of 8 mm Hg was elevated to 39 mm Hg by active shoulder flexion to 45 degrees and to 56 mm Hg by the addition of a one kilogram weight to the hand in the elevated position. Recently, morphological evidence has emerged which supports the concept of compressive loading of the supraspinatus tendon. Okuda (Okuda, Gorski, 1987) described fibrocartilaginous areas in areas of tendons subjected to compression. Riley et al (Riley, Harrall, 1994 June) found such areas in the supraspinatus tendon and noted that they had the proteoglycan/glycosaminoglycan of tendon fibrocartilage. They indicated that these morphological features were an adaptation to mechanical forces, including compression. It has been questioned whether or not compression of the cuff by the acromion could produce the type of cuff defects commonly seen in clinical practice. Recent investigations with a rat model (1996) demonstrated that increasing the loading and abrasion of the cuff tendon by the addition of bone plates between the acromion and the tendon produced only bursal side lesions and never the intratendinous or articular side cuff tendon defects which are most frequently seen clinically.

Figure 27

Figure 28

Figure 29
Although young healthy tendons seem to tolerate their complex loading situation without difficulty, structurally inferior tissue (Kumagai, Sarkar, 1994, Riley, Harrall, 1994 June), tissue with compromised repair potential (Dalton, Cawston, 1995, Hamada, Okawara, 1994), or tendons frequently subjected to unusually large loads (as in an individual with paraplegia) (Bayley, Cochran, 1987) may degenerate in their hostile mechanical environment. (Godsil and Linscheid, 1970, Ozaki, Fujimoto, 1988, Riley, Harrall, 1994)
Tendon degeneration
Normal tendon is exceedingly strong. The work of McMasters (McMaster, 1933) is frequently quoted in this regard. He conducted experiments showing that loads applied to normal rabbit Achilles tendons produced failure at the musculotendinous junction, at the insertion into bone, at the muscle origin, or at the bone itself, but not at the tendon midsubstance. In his preparation, one-half of the tendon's fibers had to be severed before the tendon failed in tension. If the tendon was crushed with a Kocher clamp, pounded, and then doubly ligated above and below the injury, rupture could be produced in half of the specimens when tested over four weeks later. Normal tendon is obviously tough stuff!
It is estimated that in normal activities, the force transmitted through the cuff tendon is in the range 140 to 200 Newtons. (Laing, 1956, Liberson, 1937, Lohr and Uhthoff, 1990, Samilson, 1980). The ultimate tensile load of the supraspinatus tendon in specimens from the sixth or seventh decade of life has been measured between 600 and 800 Newtons. (Itoi, Berglund, 1995)
While cuff strength may be compromised by inflammatory arthritis (Cofield, 1987, McCarty, Haverson, 1981) and steroids (Ellman, Hanker, 1986, Kennedy and Willis, 1976), the primary cause of tendon degeneration is aging. Like the rest of the body's connective tissues, rotator cuff tendon fibers become weaker with disuse and age; as they become weaker, less force is required to disrupt them (see figure 30). (Chung and Nissenbaum, 1975, Neer, 1978, Rathbun and Macnab, 1970, Swiontkowski, Iannotti, 1990) Hollis and associates (Hollis, Lyon, 1988) showed that the anterior cruciate ligament of a 70-year-old is only 20 to 25 per cent as strong as that of a 20-year-old. Others have shown similar loss of tendon strength with age. (Codman, 1934b, DePalma, 1973, Lindblom, 1939a, Lindblom, 1939b, Lindblom and Palmer, 1939, Macnab, 1973, Neer, 1972, Neer, 1983, Nixon and DiStefano, 1975, Pettersson, 1942, Watson-Jones, 1961) Uhthoff and Sarkar concluded that "Aging is the single most important contributing factor in the pathogenesis of tears of the cuff tendons." (Uhthoff and Sarkar, 1993)
Pettersson (Pettersson, 1942) provides an excellent summary of the early work on the pathology of degenerative changes in the cuff tendons. Citing the research of Loschke, Wrede, Codman, Schaer, Glatthaar, Wells, and others, he builds a convincing case for primary, age-related degeneration of the tendon manifested by changes in cell arrangement, calcium deposition, fibrinoid thickening, fatty degeneration, necrosis, and rents. He states that "the degenerative changes in the tendon aponeurosis of the shoulder joint, except for calcification and rupture, give no symptoms, as far as is known at present. On the other hand the tensile strength and elasticity of a tendon aponeurosis that exhibits such degenerative lesions are unquestionably less than in a normal tendon aponeurosis."
The major role of tendon degeneration in the production of cuff defects was promoted as a concept by Meyer (Meyer, 1924, Meyer, 1931) and corroborated by the studies of DePalma and others (Cotton and Rideout, 1964, DePalma, 1983, DePalma, Gallery, 1949, DePalma, White, 1950, Grant and Smith, 1948, Neer, 1990, Ozaki, Fujimoto, 1988, Uhthoff and Sarkar, 1991a, Zuckerman, Kummer, 1992). Nixon and DiStefano, reviewing the literature on the microscopic anatomy of cuff deterioration, (Nixon and DiStefano, 1975) found loss of the normal organizational and staining characteristics of bone, fibrocartilage, and tendon without evidence of repair. They summarized these degenerative changes as follows:
"Early changes are characterized by granularity and a loss of the normal clear wavy outline of the collagen fibers and bundles of fibers. The structures take on a rather homogenous appearance; the connective tissue cells become distorted and the parallelism of the fibers is lost. The cell nuclei become distorted in appearance--some rounded, others pyknotic or fasiculated. Some areas of the tendon have a gelatinous or edematous appearance with loosening of fibers that contain broken, frayed elements separated by a pale staining homogeneous material". This histological picture is reminiscent of that described for tennis elbow, Achilles tendinitis and patellar tendinitis.
Brewer (Brewer, 1979) has demonstrated age-related changes in the rotator cuff. These changes include diminution of fibrocartilage at the cuff insertion, diminution of vascularity, fragmentation of the tendon with loss of cellularity and staining quality, and disruption of the attachment to bone via Sharpey's fibers. The bone at the insertion becomes osteoporotic and prone to fracture. (Kannus, Leppala, 1995)
Recently Kumagai et al (Kumagai, Sarkar, 1994) studied the attachment zone of the rotator cuff tendons to determine how degenerative changes affected the pattern of collagen fiber distribution. Degenerative changes were found in all elderly tendons but not in tendons from younger subjects. Changes in insertional fibrocartilage included calcification, fibrovascular proliferation and microtears. In degenerative tendons, the normal distribution of collagen fiber types was markedly altered with fibrovascular tissue containing type III collagen instead of the usually predominant type II. The authors concluded that severe degenerative changes in the cuff tendons of elderly individuals alter the collagen characteristic of the rotator cuff and that the changes could be associated with impairment of biomechanical properties of the attachment zone. Virtually identical findings were reported by Riley. (Riley, Harrall, 1994)
In all clinical reports, the incidence of cuff defects is relatively rare before the age of 40 and begins to rise in the 50- to 60-year age group and continues to increase in the 70-year and over age group. Of 55 patients with arthrographically verified cuff tears, Bakalim and Pasila (Bakalim and Pasila, 1975) found only 3 who were under 40 years of age. Yamada and Evans found no cuff tears in 42 shoulders under age 40. (Yamada and Evans, 1972) In Hawkins and coworkers' series of 100 cuff repairs, only 2 patients were in their third or fourth decade. (Hawkins, Misamore, 1985) In their series of shoulder dislocations, Reeves (Reeves, 1966) and Moseley (Moseley, 1969) found the incidence of cuff tears among patients under 30 to be very low. These authors found that the incidence of cuff failure in dislocated shoulders rose dramatically with the age of the patient. As noted by DePalma, even massive injuries to young healthy shoulders "seem more likely to produce glenohumeral ligament tears and fractures than ruptures of the rotator cuff." Pettersson (Pettersson, 1942) states that "even in cases of traumatic rupture . . . the age distribution indicates that changes in the elasticity and tensile strength are prerequisites for the appearance of the rupture." Pettersson (Pettersson, 1942) reported further that in patients with anteroinferior dislocations, the incidence of arthrographically proven partial- or full-thickness cuff tears was 30 per cent in the fourth decade and 60 per cent in the sixth decade.
Many cuff defects occur in 50- to 60-year-old individuals who have led quite sedentary lives without a history of injury or heavy use. In one of his clinical series, Neer provided substantial evidence for a degenerative etiology of cuff defects:(Neer, 1983)
- 40 per cent of those with cuff defects have "never done strenuous physical work";
- cuff defects are frequently bilateral;
- many heavy laborers never develop cuff defects; and
- 50 per cent of patients with cuff defects had no recollection of shoulder trauma.
In their 1988 report to the American Shoulder and Elbow Surgeons (ASES), Neer and coworkers (Neer, Flatow, 1988) found that of 233 patients with cuff defects, all but 8 were over 40; 70 per cent of the defects occurred in sedentary individuals doing light work, 27 per cent in females, and 28 per cent in the non dominant arm.
As expected, the deterioration in cuff quality is usually bilateral: Harryman found that 55% of patients presenting with cuff tears on one side had ultrasonographic evidence of cuff defects on the contralateral side. (Matsen, Lippitt, 1994) Age related degeneration can also be observed by MRI. (Tyson and Crues III, 1993)
The pattern of degenerative cuff failure is distinctive. E.A. Codman described the "rim rent" in which the deep surface of the cuff is torn at its attachment to the tuberosity. (Codman, 1934b) Codman's wonderful book contains many photomicrographs of these rim rents, providing a convincing argument that cuff tears most frequently begin on the deep surface and extend outward until they become full-thickness defects (see figure 44). Codman pointed out that: "It would be hard to explain this . . . by erosion from contact with the acromion process." Similarly, McLaughlin (McLaughlin, 1944) observed that partial tears of the cuff "commonly involve only the deep surface of the cuff..." Wilson and Duff (Wilson and Duff, 1943) also described partial tears near the insertion of the cuff. These occurred on the articular surface, on the bursal surface, and in the substance of the tendon. Cotton and Rideout (Cotton and Rideout, 1964) also described "slight" tears on the deep surface of the supraspinatus adjacent to the biceps tendon in their necropsy studies. Pettersson and DePalma noted that the innermost fibers of the cuff begin to tear away from their bony insertion to the humeral head in the fifth decade and that these partial-thickness tears increase in size over the next several decades. (DePalma, 1973, Pettersson, 1942) The partial-thickness tears observed by Uhthoff and coworkers (Uhthoff, Loehr, 1986 Oct 27) were always on the articular side; none occurred on the bursal side in spite of the occasional presence of spurs or osteophytes on the acromion. Other authors also have described partial thickness tears. (Bosworth, 1940, Bosworth, 1941, Codman, 1934b, Fukuda, 1980, Fukuda, Mikasa, 1987, Kutsuma, Akaoka, 1982, Mikasa, 1979, Ozaki, Fujimoto, 1985, Strizak, Danzig, 1982, Tabata and Kida, 1983, Tabata, Kida, 1981, Tamai and Ogawa, 1985, Yamamoto, 1982, Yamanaka and Fukuda, 1981, Yamanaka, Fukuda, 1983) These observations suggest that the deep fibers of the cuff near its insertion to the tuberosity are most vulnerable to failure, either because of the loads to which they are exposed or because of their relative lack of strength or because of their limited capacity for repair.
An important recent study by Fukuda et al documented the patterns of intratendinous tears and observed that these lesions tend not to heal. (Fukuda, Hamada, 1994) Further evidence of the non healing of cuff lesions was provided by Yamanaka and Matsumoto(Yamanaka and Matsumoto, 1994) who demonstrated progression of partial thickness tears. After initial arthrography, they followed 40 tears (average patient age 61 years) managed without surgery. Repeat arthrograms an average of one year later showed apparent healing in only 10%, reduction of apparent tear size in 10%, and enlargement of the tear size in over 50% with over 25% progressing to full thickness tears. Interestingly the clinical pain and function scores of these patients were improved at followup. These observations lend proof to Codman's statement 60 years earlier, "It is my unproved opinion that many of these lesions never heal, although the symptoms caused by them usually disappear after a few months. Otherwise, how could we account for their frequent presence at autopsy?" (Codman, 1934b) These studies also demonstrate the critical point that scores based on clinical symptoms are an unreliable way of determining the integrity of the cuff tendon.
Pathogenesis
The traumatic and the degenerative theories of cuff tendon failure can be synthesized into a unified view of pathogenesis. Through its life the cuff is subjected to various adverse factors such as traction, compression, contusion, subacromial abrasion, inflammation, injections, and, perhaps most importantly, age-related degeneration. Lesions of the cuff typically start where the loads are presumeably the greatest: at the deep surface of the anterior insertion of the supraspinatus near the long head of the biceps (see figures 31 and 32). Tendon fibers fail when the applied load exceeds their strength. Fibers may fail a few at a time or en masse (see figure 33). Because these fibers are under load even with the arm at rest, they retract after their rupture. Each instance of fiber failure has at least four adverse effects:
- it increases the load on the neighboring as yet unruptured fibers, giving rise to the zipper phenomenon,
- it detaches muscle fibers from bone (diminishing the force that the cuff muscles can deliver),
- it compromises the tendon fibers' blood supply by distorting the anatomy contributing to progressive local ischemia (see figure 34) and
- it exposes increasing amounts of the tendon to joint fluid containing lytic enzymes which remove any hematoma which could contribute to tendon healing (see figure 35).
Even when the tendon heals, its scar tissue lacks the normal resilience of tendon and is, therefore, under increased risk for failure with subsequent loading. These events weaken the substance of the cuff, impair its function, and diminish its ability to effectively repair itself. In the absence of repair, the degenerative processtends to continue through the substance of the supraspinatus tendon to produce a full thickness defect in the anterior supraspinatus tendon (see figure 36). This full thickness defect tends to concentrate loads at its margin, facilitating additional fiber failure with smaller loads than those which produced the initial defect (see figure 37). With subsequent episodes of loading, this pattern repeats itself, rendering the cuff weaker, more prone to additional failure with less load, and less able to heal. Once a supraspinatus defect is established, it typically propagates posteriorly through the remainder of the supraspinatus, then into the infraspinatus (see figure 38).
With progressive dissolution of the cuff tendon, the spacer effect of the cuff tendon is lost, allowing the humeral head to displace superiorly (see figures 29 and 39), placing increased load on the biceps tendon. As a result, the breadth of the long head tendon of the biceps is often greater in patients with cuff tears in comparison to uninjured shoulders (see figure 40). (Ting, Jobe, 1987) In chronic cuff deficiency, the long head tendon of the biceps is frequently ruptured.
Further propagation of the cuff defect crosses the bicipital groove to involve the subscapularis, starting at the top of the lesser tuberosity and extending inferiorly. As the defect extends across the bicipital groove, it may be associated with rupture of the transverse humeral ligament and destabilization of the long head tendon of the biceps allowing its medial displacement (see figure 41). (Slatis and Aalto, 1979)
The concavity compression mechanism of glenohumeral stability (see figure 18) is compromised by cuff disease. Beginning with the early stages of cuff fiber failure, the compression of the humeral head becomes less effective in resisting the upward pull of the deltoid. Partial thickness cuff tears cause pain on muscle contraction similar to that seen with other partial tendon injuries (such as those of the Achilles tendon or extensor carpi radialis brevis). This pain produces reflex inhibition of the muscle action. In turn, this reflex inhibition along with the absolute loss of strength from fiber detachment makes the muscle less effective in balance and stability. However, as long as the glenoid concavity is intact, the compressive action of the residual cuff muscles may stabilize the humeral head (see figure 42). When the weakened cuff cannot prevent the humeral head from rising under the pull of the deltoid, the residual cuff becomes squeezed between the head and the coracoacromial arch. Under these circumstances, abrasion occurs with humeroscapular motion, further contributing to cuff degeneration (see figure 43). Degenerative traction spurs develop in the coracoacromial ligament which is loaded by pressure from the humeral head (analogous to the calcaneal traction spur that occurs with chronic strains of the plantar fascia) (see figure 39). Upward displacement of the head also wears on the upper glenoid lip and labrum (see figure 44), reducing the effectiveness of the upper glenoid concavity. Further deterioration of the cuff allows the tendons to slide down below the center of the humeral head, producing a "boutonniere" deformity (see figure 41). (Norris, Fischer, 1983) The cuff tendons become head elevators rather than head compressors. Just as in the boutonniere of the finger, the shoulder with a buttonholed cuff is victimized by the conversion of balancing forces into unbalancing forces. Erosion of the superior glenoid lip may thwart attempts to keep the humeral head centered after cuff repair (see figure 42). Once the full thickness of the cuff has failed, abrasion of the humeral articular cartilage against the coracoacromial arch may lead to a secondary degenerative joint disease known as cuff tear arthropathy (see figures 39 and 47). (Neer, Craig, 1983)
The cuff muscle deterioration which inevitably accompanies chronic cuff tears is one of the most important limiting factors in cuff repair surgery. Atrophy, fatty degeneration, retraction, loss of excursion are all commonly associated with chronic cuff tendon defects. (Leivseth and Reikeras, 1994, Nakagaki, Tomita, 1994) To a large extent, these factors are irreversible.(Goutallier, Postel, 1994) These changes increase with the duration of the tear and do not rapidly reverse after cuff repair. (Goutallier, Postel, 1995)

Figure 30

Figure 31

Figure 32
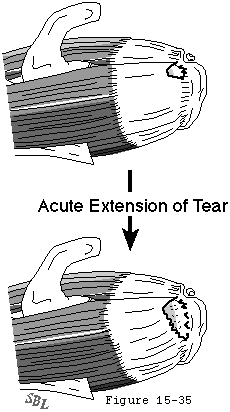
Figure 33

Figure 34

Figure 35

Figure 36

Figure 37
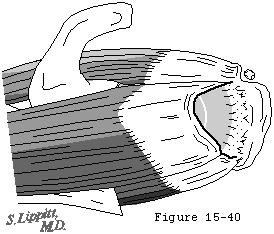
Figure 38

Figure 39

Figure 40

Figure 41

Figure 42

Figure 43

Figure 44

Figure 45

Figure 46
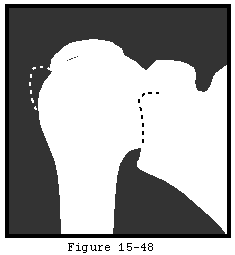
Figure 47